http://www.asyura2.com/12/jisin18/msg/337.html
Tweet |
(回答先: 太陽でも超巨大爆発「スーパーフレア」京大解析、通説に疑義 多数発見=爆発現象、太陽の数千倍も 投稿者 MR 日時 2012 年 5 月 17 日 14:14:59)
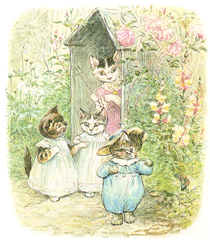

http://www.nature.com/nature/journal/vaop/ncurrent/full/nature11194.html
Astrophysics: Startling superflares
Bradley E. Schaefer
Nature (2012) doi:10.1038/nature11194
Published online 16 May 2012
Stars that are just like our Sun have flares more than a million times more energetic than the biggest flare ever seen on the Sun. The Kepler satellite has allowed these superflares to be studied in detail for the first time.
Article tools
print
email
download pdf
view in readcube
download citation
order reprints
rights and permissions
share/bookmark
A superflare on a Sun-like star is a brightening that has an energy of from 1033 to more than 1039 erg and lasts from minutes to days. The Sun has frequent flares that are caused by magnetic effects above sunspots, regions that are cooler than the Sun's typical surface temperature. However, the largest flare ever observed1 on the Sun ― the 1859 Carrington event ― had a total energy of about 1032 erg. With Sun-like stars being the epitome of constancy, it is startling, evocative and exciting that they can have superflares as energetic as 1039 erg. In a paper published on Nature's website today, Maehara et al.2 report the emissions from 365 superflares, measured by the awesome Kepler satellite, which was launched in 2009.
Over the past 120 years, four dozen superflares have been reported in the literature3, 4, 5. But these events were always ignored as isolated anomalies. Only in 1989 were all these reports put together in recognition that the events represent a coherent phenomenon, extending to all types of normal stars3, 4, 5, and the name superflares was coined to distinguish them from ordinary flares such as those that happen on the Sun5.
Superflares occur on single, middle-aged stars that are rotating slowly and are powered by the fusion of hydrogen in their core. Such stars, which are technically known as main-sequence stars of spectral type F8 to G8, include the closest known 'twins' of our Sun. The similarity of superflares to solar flares suggests that superflares arise from magnetic effects. However, the now-default model6, 7, 8, 9 for these events involves a magnetic field that connects the star to an orbiting 'hot Jupiter' ― a planet that has a mass comparable to, or larger than, that of Jupiter but that is much closer to its host star than Jupiter is to the Sun (Fig. 1).
Figure 1: Magnetic connection.
One idea to explain the superflares observed by Maehara et al.2 invokes the presence of intense magnetic fields that connect the star with a Jupiter-like planet in very close orbit around the star. The magnetic-field lines will become twisted and amplified by the orbital motion of the planet, and at some time the lines will be strained and twisted to the point of breaking. The broken lines will accelerate particles to very high energy and release this energy in an explosive event, similar to what happens in ordinary solar flares seen on the Sun.
Full size image (36 KB)
The limitation of pre-Kepler observations is that they are an inhomogeneous collection of data. Some data are from X-ray satellites, whereas others are from spectroscopic observations, multicolour photometry and even visual observations. Such an assorted data set makes it impossible to calculate rates of occurrence, or to seek correlations between observed features or do other statistical analyses. With the average recurrence timescale for the brightest events being much longer than a decade, it was unfeasible to set up any realistic programme to observe superflares, and so the field stagnated.
Maehara and colleagues2 now break this deadlock by analysing data from the Kepler satellite, which provides continuous monitoring of the brightness of more than 100,000 stars for years on end with an accuracy of 10 parts per million. The authors have looked at some 83,000 G-type main-sequence stars and provide light curves ― plots showing the evolution of an object's brightness over time ― for 365 superflares on 148 stars. Suddenly, we have a wealth of data, we can do statistical analyses, and we know exactly which stars to watch.
The Kepler superflares have durations of 1–12 hours, brightness increases of 0.1–30% and total energies of 1033−1036 erg. A typical star in the Kepler data set has 1035-erg flares every 100 days. Interestingly, Maehara et al. find that the observed superflares all occur on stars that have large starspots, as evidenced by quasi-periodic modulation of the star's quiescent brightness. This finding crucially ties the superflares to starspots and hence to magnetic fields. Another insight is that the superflare stars apparently have no planetary transits ― that is, no planets have been found to pass in front them. However, roughly 10% of the superflare stars should have such transits if they are all associated with hot Jupiters. So the mechanism underlying superflares remains unclear.
With Maehara and colleagues' results, theorists have a rich field to investigate. And I can think of many new paths that have been opened up for observers. Measurement of the radial velocity of the superflare stars might reveal Jupiter-like planets. The brightest of the superflare stars are bright enough to allow the stars' magnetic fields to be determined. High-resolution spectra of the most active stars (which have superflares every 9 days) could be obtained with a view to detecting changes in the shape of the calcium spectral lines (the H and K lines) simultaneous with a Kepler superflare. Such changes would provide information about the velocity, temperature and energy of the superflares. The Kepler data could be further scrutinized to investigate whether flares occur in preferred phases of the star's brightness modulation and whether the energies and time intervals of successive flares are correlated. Maehara and colleagues' work could also be extended to stars of all types. Indeed, this task of searching for flares is perfect for pursuits such as the Planet Hunters programme of citizen science, which is part of the Zooniverse project10.
The possibility that the Sun has superflares is not realized. Historical and geophysical records show that the Sun has not had any superflares in the past two millennia, and no superflares with more than roughly 1036 erg for perhaps a billion years5. Maehara et al. show that only 0.2% of Sun-like stars have superflares, so it is unlikely that the Sun has such events. With their average rate of occurrence (once every 100 days for 1035-erg flares) and their observed size distribution (with a power-law index of roughly −2.0), the expected frequency of 1032-erg flares on all superflare stars should be very high. In stark contrast to this, the Sun has one 1032-erg event roughly every 450 years1, 11 and so is completely different from superflare stars. This is all readily understood within the default model, because the Sun does not have a hot Jupiter.
Superflares have implications far beyond being just a challenge for stellar physics. If a superflare's energy is linked to the orbital energy of a hot Jupiter, then three events a year on the star would make its planetary companion spiral in towards it on a timescale of a billion years. The huge energy output of superflares could make any planets around the star uninhabitable for far-future human colonization, and astrobiologists will have to consider the effect of the superflares on possible alien life. Superflares might provide the high-energy radiation required to create organic molecules, so perhaps superflare systems are a good place to look for alien life that has evolved to avoid the effects of the huge flares.
References
Author information
References Author Information
Affiliations
Bradley E. Schaefer is in the Department of Physics and Astronomy, Louisiana State University, Baton Rouge, Louisiana 70803, USA.
Corresponding author
Correspondence to: Bradley E. Schaefer
http://www.nature.com/nature/journal/vaop/ncurrent/full/nature11063.html
Superflares on solar-type stars
Hiroyuki Maehara, Takuya Shibayama, Shota Notsu, Yuta Notsu, Takashi Nagao, Satoshi Kusaba, Satoshi Honda, Daisaku Nogami & Kazunari Shibata
AffiliationsContributionsCorresponding author
Nature (2012) doi:10.1038/nature11063
Received 13 October 2011 Accepted 14 March 2012 Published online 16 May 2012
Article tools
print
email
download pdf
view in readcube
download citation
order reprints
rights and permissions
share/bookmark
Solar flares are caused by the sudden release of magnetic energy stored near sunspots. They release 1029 to 1032 ergs of energy on a timescale of hours1. Similar flares have been observed on many stars, with larger ‘superflares’ seen on a variety of stars2, 3, some of which are rapidly rotating4, 5 and some of which are of ordinary solar type3, 6. The small number of superflares observed on solar-type stars has hitherto precluded a detailed study of them. Here we report observations of 365 superflares, including some from slowly rotating solar-type stars, from about 83,000 stars observed over 120 days. Quasi-periodic brightness modulations observed in the solar-type stars suggest that they have much larger starspots than does the Sun. The maximum energy of the flare is not correlated with the stellar rotation period, but the data suggest that superflares occur more frequently on rapidly rotating stars. It has been proposed that hot Jupiters may be important in the generation of superflares on solar-type stars7, but none have been discovered around the stars that we have studied, indicating that hot Jupiters associated with superflares are rare.
Figures at a glance
left
right
Main
Main References Acknowledgements Author Information Supplementary Information
We searched for stellar flares on solar-type stars (G-type main-sequence stars) using data collected by NASA’s Kepler8 mission during the period from April 2009 to December 2009 (a brief summary of our flare search method is described in the legend of Fig. 1 and more detail is provided in Supplementary Information). We used the effective temperature (Teff) and the surface gravity (log(g)) available from the Kepler Input Catalog9 to select solar-type stars. The selection criteria are as follows: 5,100 K ≤ Teff < 6,000 K, log(g) ≥ 4.0. The total numbers of solar-type stars are 9,751 for quarter 0 of the Kepler mission (the length of observation period is about 10 d), 75,728 for quarter 1 (33 d), 83,094 for quarter 2 (90 d) and 3,691 for quarter 3 (90 d).
Figure 1: Light curve of typical superflares.
a, Light curve of superflares on the G-type main-sequence star KIC 9459362. The individual points represent the difference between the observed brightness during each cadence and the average brightness during the observation period. The typical photometric error is about 0.02%. BJD, barycentric Julian date. b, Enlarged light curve of a superflare observed at BJD 2,454,993.63. The relative flux (ΔF/Fav) and the duration of this flare are respectively 1.4% and 3.9 h, which correspond to a peak bolometric luminosity of 1.3 × 1031 erg s−1 and a total energy of 5.6 × 1034 erg. c, Same as a, but for the G-type main-sequence star KIC 6034120. d, Same as b, but for the superflare that occurred on KIC 6034120 at BJD 2,455,055.22. The relative flux and the duration of this flare are respectively 8.4% and 5.4 h, which correspond to a peak bolometric luminosity of 6.8 × 1031 erg s−1 and a total energy of 3.0 × 1035 erg. These superflares were detected by the following procedure. First we analysed the long-cadence calibrated flux (time resolution, 29.4 min) and calculated the distribution of brightness changes between all pairs of consecutive data points. Then the threshold of the flare was determined to be three times the value in the top 1% of the distribution. The threshold value is typically about 0.1% of the brightness of the star. Finally we defined as flare candidates those data points at which the brightness change exceeded the threshold. Because many of these flare candidates are false events (for various reasons), for confirmation we examined their durations and the shapes of their light curves, checked for the existence of nearby stars and studied the charge-coupled-device pixel-level data for each flare candidate.
Full size image (128 KB)
Download PowerPoint slide (629K)
Figures index
Next figure
We found 365 superflares (flares with energy >1033 erg) on 148 solar-type stars (light curves of each flare are summarized in Supplementary Fig. 8 and properties of each flare star are listed in Supplementary Table 1). The durations of the detected superflares are typically a few hours, and their amplitudes are generally of order 0.1–1% of the stellar luminosity. The bolometric luminosity and total bolometric energy of each flare were estimated from the stellar radius, the effective temperature in the Kepler Input Catalog, and the observed amplitude and duration of the flare by assuming that the spectrum of white-light flares can be described by blackbody radiation with an effective temperature of 10,000 K (ref. 10). We considered the spectral response of the Kepler photometer in doing our luminosity and energy calculations. The bolometric luminosity of superflares on G-type main-sequence stars ranges from 9 × 1029 to 4 × 1032 erg s−1, and the total bolometric energy of superflares ranges from 1033 to 1036 erg (hereafter the luminosities and energies of flares given are the bolometric values). The uncertainties in the luminosities and energies of flares are estimated to be about ±60%.
The brightnesses of the two stars in Fig. 1 show quasi-periodic variation. The period of the variation of KIC 9459362 is 12.5 d and that of KIC 6034120 is 5.7 d. The brightness variation can be caused by one of the following mechanisms: rotation of a star with starspots11, orbital motion of a binary system12, eclipse by an accompanying star12 or stellar pulsation13. Here stellar pulsation can be excluded because the pulsation period of the G-type main-sequence stars is shorter than a few hours13. The possibility of the brightness variation being due to rotation must be carefully distinguished from its being due to orbital motion, on the basis of the difference in the shape of the light curves. The variability type of KIC 9459362 and KIC 6034120 is classified as stellar rotational modulation14. The energy of superflares can be explained by the magnetic energy stored near starspots. If the time variations in the brightnesses of KIC 9459362 and KIC 6034120 are caused by their rotation and the existence of starspots, their brightness variations suggest that solar-type stars with superflares have much larger starspots than does the Sun (for more details on the relation between starspots and superflares, see Supplementary Information). Typical periods of brightness variation range from one day to a few tens of days. According to the results of the variability-type classification14, about 65% of the brightness variations in the quarter-1 data can be taken to result from stellar rotational modulation. Although we could not exclude the possibility of the binary-eclipse scenario by using only photometric data, we assumed that the period of quasi-periodic modulation corresponds to the period of stellar rotation (for more details on the possibility of superflares on secondary stars, see Supplementary Information).
The average occurrence frequency of superflares can be estimated from the number of observed superflares, the number of observed stars and the length of the observation period. For example, in the case of slowly rotating G-type main-sequence stars with surface temperatures of 5,600 K ≤ Teff < 6,000 K, 14 superflares were detected from the data on about 14,000 stars over 120 d. Hence, the occurrence frequency of superflares is 2.9 × 10−3 flares per year per star, which corresponds to a superflare occurring on a star once every 350 yr. The occurrence frequency distribution of superflares on solar-type stars can be fitted in the energy range ≥4 × 1034 erg using a simple power law (Fig. 2a, b). The frequency distribution function of superflares on solar-type stars is similar to those of solar flares15 and stellar flares on red dwarfs16. The power-law index of the distribution of superflares (−2.0 to −2.3) is nearly equal to that of the distribution of solar flares. The occurrence frequency of superflares on slowly rotating G-type main-sequence stars is about ten times lower than the average occurrence frequency (Fig. 2b). The occurrence frequency also depends on the surface temperature of the star (Fig. 2c, d), and is higher for lower-temperature (5,100 K ≤ Teff < 5,600 K) G-type main-sequence stars than for higher-temperature (5,600 K ≤ Teff < 6,000 K) stars. The superflares of 1034 erg on Sun-like stars (that is, slowly rotating G-type main-sequence stars with surface temperatures of 5,600 K ≤ Teff < 6,000 K; Fig. 2d) occur once every 800 yr, and those of 1035 erg occur once every 5,000 yr, although accurate statistics are difficult to obtain because only 14 superflares have been observed on Sun-like stars.
Figure 2: Frequency distribution of superflares on G-type main-sequence stars.
a, Number distribution of flares as a function of observed flare amplitude. The errors were estimated from the 10% uncertainty in the amplitude and the square root of the event number in each bin. The number of flares (N) is 365. b, Frequency distribution of flares as a function of flare energy. The vertical axis indicates the number of superflares per star per year per unit energy. The histograms show the frequency distribution of flares on all G-type main-sequence stars (solid line) and on G-type main-sequence stars excluding the stars that have quasi-periodic brightness modulations with periods shorter than 10 d (dashed line). The error bars represent the 1σ uncertainty in the frequency estimated from the uncertainty in the energy estimation and the square root of the event number. The occurrence distribution of superflares can be fitted by a simple power-law function in the large-energy regime between 4 × 1034 and 2 × 1036 erg. The power-law indexes of the distributions are −2.3 ± 0.3 and −2.0 ± 0.2, respectively (downward-sloping lines). Because the detection completeness of flares with energy less than 4 × 1034 erg is low (10−3 for 1033 erg and 10−1 for 1034 erg), we did not use these data for the fitting. Because of the small number of events in the energy bins above 1036 erg, the fit does not well represent the data. c, Same as b, but for flares on all lower-temperature (5,100 K ≤ Teff <5,600 K; solid line) stars and all higher-temperature (5,600 K ≤ Teff < 6,000 K; dashed line) stars. d, Same as c, but only for flares on slowly rotating stars (that is, excluding stars showing brightness variations with periods shorter than 10 d).
Full size image (101 KB)
Download PowerPoint slide (482K)
Previous figure
Figures index
Next figure
As shown in Fig. 3a, the maximum energy of a superflare does not show any clear correlation with the period of stellar rotation, assuming that the period of brightness modulation corresponds to the rotational period of the star. If the flare energy can be explained by the magnetic energy stored near the starspot11, this result suggests that the maximum magnetic energy stored near the spot does not have a strong dependence on the period of rotation. This result also implies that superflares can occur on slowly rotating solar-type stars like the Sun.
Figure 3: Relations between the brightness variation period and the properties of flares on G-type main-sequence stars.
a, Scatter plot of the flare energy as a function of the variation period. An apparent negative correlation between the variation period and the lower limit of the flare energy is caused by the detection limit of our flare-search method. The vertical bar in the panel indicates the typical uncertainty in the flare energy. b, Distribution of the occurrence of flares in each period bin as a function of variation period. The vertical axis indicates the number of flares with energy ≥5 × 1034 erg per star per year. The error bars represents the 1σ uncertainty estimated from the uncertainty in the energy estimation and the square root of the event number in each period bin.
Full size image (86 KB)
Download PowerPoint slide (467K)
Previous figure
Figures index
The frequency of superflares tends to decrease as the period increases to periods longer than a few days (Fig. 3b). The frequency of superflares on the slowly rotating stars (rotational period, >10 d) is only 1/20 of that of superflares on rapidly rotating stars. The rotation period correlates with the chromospheric activity, which is known to be an indicator of the magnetic activity of the stars17, and the more rapidly rotating stars have higher magnetic activity18. According to the dynamo theory of magnetic field generation, magnetic activity results from the interaction between rotation and convection19, and the rapid rotation can cause the high magnetic activity. Our result implies that rapidly rotating stars with higher magnetic activity can cause more frequent superflares. The frequency distribution of superflares saturates for periods of less than a few days. A similar saturation is known for the relationship between the coronal X-ray activity and the rotation period20.
The rotation period of a star is also known to be related to the star’s age, with younger stars rotating more rapidly21, 22. Our findings suggest that superflares occur more frequently on the solar-type stars younger than the Sun. Moreover, on solar-type stars similar in age to the Sun, superflares occur less frequently but are nearly equal in energy to the superflares on the younger stars.
It has been pointed out that there is no record of solar superflares over the past 2,000 yr (ref. 3). According to the measurement of the impulsive nitrate events in polar ice, the largest proton flare event during the past 450 yr is the Carrington event23, which occurred on 1 September 185924. The total energy released in this flare was estimated to be of order 1032 erg (ref. 25), which is only 1/1,000 of the maximum energy of flares on slowly rotating Sun-like stars. It has also been proposed that hot Jupiters have an important effect on stellar magnetic activity7, 26, 27 and that superflares occur only on solar-type stars with hot Jupiters. However, there is no hot Jupiter in the Solar System. For these reasons, it was suggested that a superflare on the Sun is extremely unlikely3, 7. Although the Kepler mission has discovered 1,235 exoplanet candidates around 997 host stars from a survey of 156,453 stars28, no exoplanet has been found around the 148 G-type main-sequence stars with superflares. For a solar-type star with a hot Jupiter, the probability of a transit of the planet across the star is about 10% averaged over all possible orbital inclinations. If the superflares on all 148 stars were caused by hot Jupiters, then Kepler should detect 15 of them from transits. However, the Kepler planetary-transit search is almost complete for hot Jupiters29, and the non-detection of planetary transits therefore suggests that hot Jupiters associated with superflares are rare.
References
Acknowledgements
Author information
Main References Acknowledgements Author Information Supplementary Information
Affiliations
Kwasan and Hida Observatories, Kyoto University, 17 Ohmine-cho Kita Kazan, Yamashina-ku, Kyoto City, Kyoto 607-8471, Japan
Hiroyuki Maehara, Takuya Shibayama, Shota Notsu, Yuta Notsu, Takashi Nagao, Satoshi Kusaba, Satoshi Honda, Daisaku Nogami & Kazunari Shibata
Contributions
H.M. was responsible for planning, coordination and data analysis. He also wrote the majority of the text. T.S. performed the data analysis for many stellar flares observed by Kepler, and S.N. and Y.N. analysed the rotation of stars observed by Kepler. T.N. and S.K. helped with data analysis and interpretation. S.H. and D.N. contributed to the interpretation of the stellar brightness variation and helped with the data analysis from a general stellar astronomical point of view. K.S. provided theoretical interpretation of the observations and gave advice on the paper’s content.
Competing financial interests
The authors declare no competing financial interests.
Corresponding author
Correspondence to: Hiroyuki Maehara
Supplementary information
この記事を読んだ人はこんな記事も読んでいます(表示まで20秒程度時間がかかります。)

すべてのページの引用、転載、リンクを許可します。確認メールは不要です。引用元リンクを表示してください。